Correlating the Orientation of Carolina bays to a Cosmic Impact
Introduction
Researchers generally consider the bays to be formed within or excised from pre-existing strata (Prouty, 1952; Eyton & Parkhurst, 1975]. We propose that the bays are surface imperfections within a blanket of ballistically deposited shattered sedimentary strata (sand), generated at time of emplacement by the energetic deflation of steam inclusions. The authors feel this interpretation explains many of the bays’ physical characteristics, such as companion bays at different elevations, occasionally intersecting or overlaying one another. Also explained is a mechanism to create bays on ridges, the ridges themselves being composed of ejecta deposition, which is our interpretation of the Goldsboro Ridge in NC [Daniels, R.B., and E.E. Gamble, 1970].
The lack of a correlated impact structure in North America is problematic for any attempt to implicate a cosmic impact in the genesis of the Carolina bays. Our hypothesis holds that the impacting object was a massive low-density hydrated silicate object, likely a cometary body, which impacted the earth on a shallow angle, nearly tangential to the earth’s surface. Remote sensing has show that approximately 5% of all craters are created during such oblique impacts, creating a set of recognizable characteristics: oval shape, butterfly ejecta pattern, “no-fly” ejecta area up field, and “blow-out” rim down field. [Herrick, R.R, 2009; Herrick R.R. and K. Hessen, 2003]. Recent studies suggest that impacts into solid surfaces protected by a layer of low impedance materials generate structures that differ from the classic crater planforms [Schultz, P. H. and A. M. Stickle, 2009]. In our specific case, we invoke the Wisconsinan ice shield as a low-impedance layer protecting the sedimentary strata of the Michigan basin.
An ejecta curtain wall radiating outward from a cosmic impact should follow a few simple laws of large-scale geophysical flows. We discuss our analytical model engineered to replicate those flows in the Analytical Modeling section.
Using the facilities and satellite imagery of Google Earth’s GIS, augmented with high resolution digital elevation mapping (DEM) data from the USGS (Figure 1), a survey was undertaken to catalogue the full extent of Carolina bays, indexed as localized “fields” of bays. This catalogue is available on line through the use of the Google Earth GIS and a Keyhole Markup Language (kml) file you can retrieve from the web page at Carolina Bay Survey.
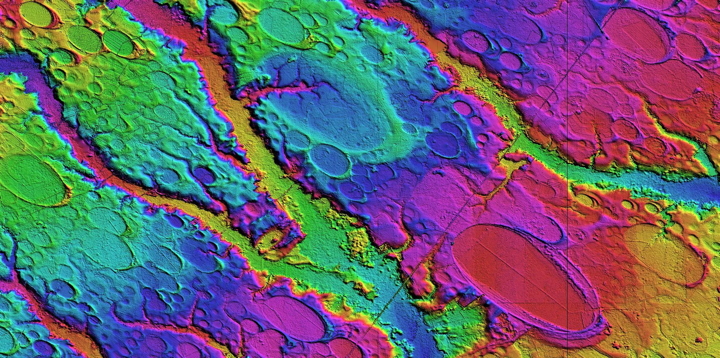
Figure 1 - LiDAR DEM Graphic of Bays in North Carolina (linked to higher resolution image)
An analytical model was developed to predict the expected trajectories and emplacement orientation characteristics of the hypothetical ejecta blanket. The model was then employed heuristically to correlate its algorithmically predicted arrival bearing against the empirically measured major axis bearings across the entire geographical extent of Carolina bay landforms in our catalogue. We present here our evidence that a unique combination of impact location and average ejecta curtain wall velocity will produce a satisfactory orientation prediction at all catalogued bay locations.
The numerical model was extended to "reverse" the geophysical flows, such that the empirically measured inferred arrival bearing could be used to drive a "walk-back" bearing towards the punitive impact site. A triangulation network was built from all catalogued fields to validate the impact site identification(Figure 2).
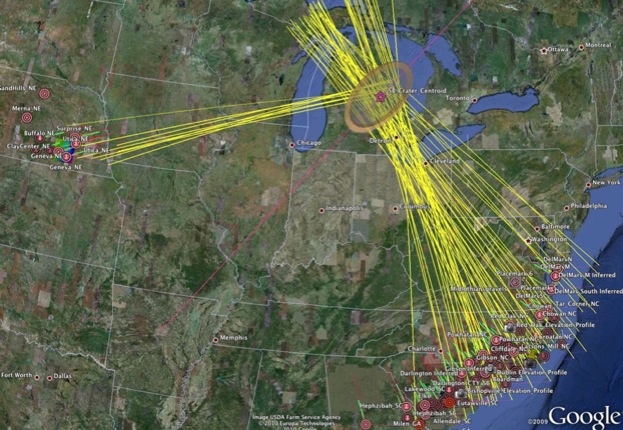
Figure 2 - Saginaw Bay Impact Triangulation Using Numerical Model "Walk-Back"
This heuristic process has identified the Saginaw area of Michigan’s Lower Peninsula (LP) as the impact site. While the area does not present classic impact crater characteristics, it does correlate to our hypothetical impact, which is modeled as an oblique event that generates a shallow oval crater (Figure 3) . Further, we implicate the presence of the multi-kilometer thick Laurentide ice sheet at the impact site during the proposed manifold date of ~780 ka, at the time of the Mid-Pleistocene Revolution. The ice sheet offers a rationale for the relatively shallow "crater" seen in the area today, while at the same time providing the significant volume of water necessary to create the posited hydrated slurry. Implicating the ice sheet also provides a vehicle to re-distribute the local crater ejecta across a wide area as "glacial till" during the succeeding seven 100ka-cycle ice age advances. This “drift” has been comfortably evaluated by others in a gradualistic glacial context.
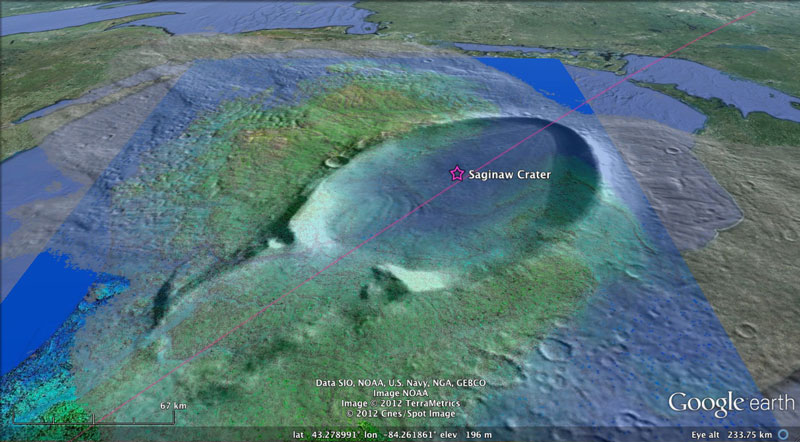
Figure 3 - Proposed Saginaw Crater with an Example Mars Oblique Impact Crater Overlaid in Google Earth
2.0) Context & History
In our 2006 AGU submissions (AGU 2006 T41A-03) and its referenced web-based documentation here on Cintos.org, we presented our case that an extraterrestrial impact occurred into the North American Wisconsinian Ice sheet. Recent work by others (Firestone, et al) added significant support to such a hypothesis.
We presented a poster (PP31D-1387) at the December 2009 AGU Fall Meeting in San Francisco which detailed some of our concepts as they pertain to the Carolina bays and their role in identifying a possible ice shield impact crater. A PDF file of the submission in slide presentation form is available for download HERE. Links are available to two videos on You Tube (Part 1& Part 2) in which we discuss the poster at the AGU meeting. Please note that since the meeting, we refined the impact location to the Saginaw Bay area of Michigan, and that constraints have been identified while dating various Carolina bay structures which had lead us more recently to a proposed date of ~150,000 years ago, well before the Younger Dryas. We now fix the data explicitly at the time of the massive cosmic impact that produced the Australasian Tektite strewnfield - ~785,000 years ago. More massive than the 35 million year old Chesapeake Bay impact (85km diameter), and 40 times more recent!
Our efforts and hypothesis diverge from the Firestone group in several major areas. We suggest that the entire assemblage of sand distributed within any given Carolina bay structure is ejecta, not simply a few scattered grains. We propose that a massive crater is existent, as necessary to derive such an extensive ejecta blanket.
2.1) Ovoid Shapes Are Generated in Splatter Formations
In a series of experiments, simulated ejecta of various sizes and composition were propelled onto a flat surface, impacting at shallow angles. The “droplets” were transformed into oval-shaped geometries.- Parameters affecting the geometric shape of ejecta “bays” :
- Angle of incidence: shallower angles will yield a higher length to width ratio
- Hydration ratio of slurry: wetter mixes slump more than stiffer, dryer material
- Host bed: flat surfaces vs. complex terrain determine veneer retention
- Size of cavity: Effect scales linearly, with child bays seen within parent bodies
Ejecta as a sheet would generate a field of varying sizes bays in general alignment. Large emplacements have bays imbedded in them, possibly due to varying density regions in the slurry droplet. Geometry scales to kilometers in size.
These characteristics are tightly constrained by the formative processes and circumstances, resulting in a simple, well-defined and unique set of identification criteria to identify these formations. The stylistic geometry and anomalous, unstratified, and homogeneous nature of these landforms are seen as being most indicative of set membership. The samples in Figures 4a and 4c show some of the experimental results obtained during our research contrasted with an actual bay (Figure 4b)
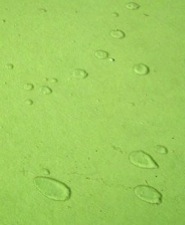
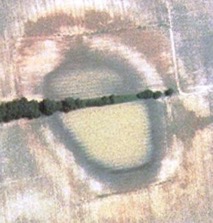
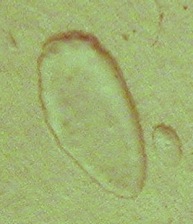
Figures 4a, b, c - Samples of splatter produced by experiment compared to a Carolina bay
2.2) Carolina bays Interpreted as Depositional Artifacts in a Veneer of Ejecta
While interpretation of the Carolina bays sands as distal ejecta structures would be controversial due to its dependence on a truly massive deposition of ejecta, the creation of the stylized Carolina bay geometry can easily be seen in the simple paint splatter. Others have probed and researched the bays and their contents, but those investigations have always considered that the bays were formed within or excised from a hosting strata of sand or gravel which itself was created by well-understood fluvial and eolian deposition [Eyton, J.R. and Parkhurst, 1975]. In our interpretation, as much as a 10-meter thick “sheet” of ejecta slurry from the central Michigan impact overlay the region. The bays are a surface feature of that veneer of strata. This interpretation helps to explain the creation of bays seen at significantly varying elevation while being quite close to each other, often even overlaying each other. Also explained is a mechanism that could create bays on ridges; the ridges themselves being comprised of ejecta deposition. This is our interpretation of the structure know as the Goldsboro Ridge in NC. The Distal Ejecta section of the Saginaw Manifold discusses this further.The Distal Ejecta section of the Saginaw Manifold discusses this further.
We suspect that the very shallow planform is driven by its genesis as a "popped bubble" as high pressure steam inclusion in the ejecta blanket are deflated upon emplacement.
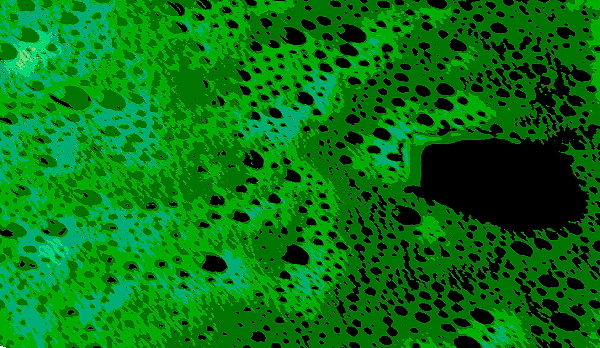
Experimental Results using soap foam and graphical editing
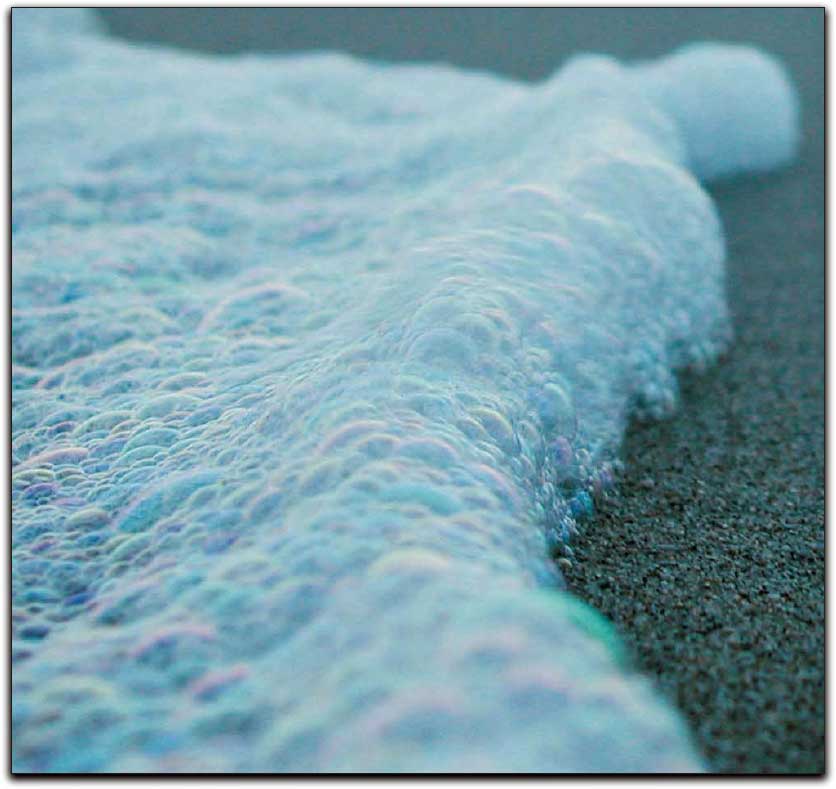
Foamy Wave of bubbles
2.3) Oblique Impact Crater Characteristics
A challenging aspect of any attempt to implicate a cosmic impact in the genesis of the Carolina bays involves the lack of an identifiable impact structure. Our conjecture holds that the impacting object was a hydrated silicate object, likely a cometary body, which impacted the earth on a shallow angle, nearly tangential. We are considering an object striking the earth out of solar orbit, or one that was traveling in a terrestrial earth orbit for a time prior to the impact. Given the relatively the shallow angle of incidence and the hydrated nature of a cometary impactor, the geological signature of the proposed impact structures is unconventional: both shallow and oval.
Imaging of the surfaces of solar system terrestrial planets and moons has show that approximately 5% of all craters are created during low angle of incidence - oblique - impacts. These events create a set of recognizable characteristics: oval shape, butterfly ejecta pattern, "no-fly" ejecta area up field, and "blow-out" rim down field, as displayed in Figure 5a.
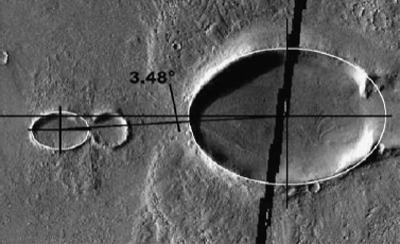
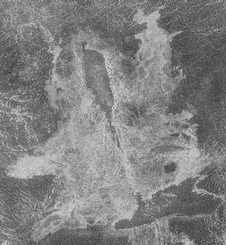
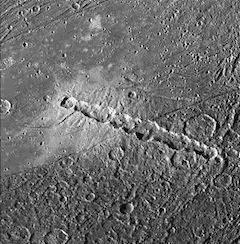
Figure 5a -Oval Impact Crater, Figure 5b-Butterfly Ejecta Planform, and Figure 5c - Chain-of-Craters Examples
Oblique impacts have shown to generate ejecta in a stylized “Butterfly” pattern (Figure 5b), spreading laterally from along the major axis of the oval crater. In the specific case of the ice-sheet covered impact area, excised terrestrial minerals, along with cometary minerals and glacial ice would be intermixed and distributed as a wave of ejecta. Velocities of the ejecta are seen as being 25-50% of the impactor’s, ~2 to 4 km/sec, yielding ejecta fields beyond 1000km downrange. We call special attention to the work of Schultz and Stickle [Lost Impacts, 2009], which explains how shallow angle of incidence (oblique) impacts into solid surfaces protected by a layer of low impedance materials generate "impact" structures that are significantly different from the classic, better understood, crater planforms.
Our proposed impactor, being delivered out of terrestrial orbits, would generate impacts at angles of less than 5 degrees - nearly tangential. The crater generated would manifest itself as a quite shallow and elongated oval. A comet captured into a terrestrial orbit would be subjected to tidal forces when it comes within the Roche radius. This often fragments the progenitor body into a string of impactors (Figure 5c). The SL-9 event demonstrated the concept, and train crater impact signatures have been seen on several planets and moons, including our own. Alternatively, a glancing impact directly from a solar orbiting body is also being considered, although the impact energies would be considerably higher.
3.0) Methods
3.1) Determining the Geographical Extent of Carolina bay landforms
Using the facilities and satellite imagery of Google Earth’s GIS, augmented with high resolution digital elevation mapping (DEM) data from the USGS, a survey was undertaken to catalogue the full extent of Carolina bays, indexed as localized “fields” of bays. This catalogue is available on line through the use of the Google Earth GIS and a Keyhole Markup Language (kml) file entitled Distal_Ejecta_Fields. This file contains placemarks with metadata annotations about the field, a "portrait" of the field, and a link to a set of kml specific to that field.
Finding Carolina bays on the costal plain of the Carolinas is easy, given their plentiful quantities and solid identification, but as the ejecta butterfly arc is walked northerly and southerly, the search becomes more challenging. Some of this is due to the more rounded shapes seen above Maryland and down in Georgia. An additional challenge is the increasingly rough terrain seen when moving inland from the costal plains. Our proposed ejecta blanket sheet model suggests that the bays are present as defects (popped bubbles) in a thin layer of sandy ejecta. These can persist over the thousands of years of erosion only under special circumstances. If the landing area is relatively flat and moist, they will be easily stabilized as bays. If the area is level but very dry, the blanket will be reworked by the wind into a generic dune field, obliterating any bay formation.
When the landing field is in rough terrain, we propose it is quickly sloughed off in erosion. Any bay formations that are draped over elevations that exceed the bay's rim height cannot hold moisture for stabilization. Thus in hilly areas further inland on the east coast, we have pursued the search for bays by identifying areas which exhibit level terrain. These can usually seen as plateaus in the digital elevation maps (DEMs) we are using. The plateaus could be indications of a plateau extent present when the ejecta landed, or be merely the surviving remnants of a larger plateau that has been invaded by erosional valleys. When the landing field is in rough terrain, we propose it is quickly sloughed off in erosion. Any bay formations that are draped over elevations that exceed the bay's rim height cannot hold moisture for stabilization. Thus in hilly areas further inland on the east coast, we have pursued the search for bays by identifying areas which exhibit level terrain. These can usually seen as plateaus in the digital elevation maps (DEMs) we are using. The plateaus could be indications of a plateau extent present when the ejecta landed, or be merely the surviving remnants of a larger plateau that has been invaded by erosional valleys. In the latter case, we see that encroaching erosional valleys later compromised bays, which once were stabilized.
While there is a great deal of research covering the "Carolina bays" on the eastern seaboard of the US, little attention has been paid to the significant quantity of oval-shaped landforms in the eastern areas of Nebraska. These, too, are aligned with each other. Significantly, the inferred alignment is considerably different from that of the background predominate-wind orientation of the extensive sand dunes in the area. Our interpretation is that the "inferred alignment" of all the Nebraskan bays we have identified are aligned towards the Saginaw impact site, and are correlated to a high degree using the Bearing Calculator tool.
While not nearly as extensive as those in the east, we hold the identification of these bays to be critical to the impact site triangulation process, as contrasted with their eastern-US brethren. A relationship between the Rainwater Basins of Nebraska and the Carolina bays was first described by William Zaner (GSA Annual Meeting, 2001). George Howard was the first to web-publish high resolution LiDAR images of bays in Nebraska.
3.2) A Heuristic Argument
Our methodology for determining the origin of the posited "ejecta" has been heuristic. The Inferred alignments of ~40 Carolina bay fields were triangulated, and a geometric centroid was determined by averaging the first pass triangulation points. Using that centroid and the Systematic by Loft Time conjecture, an east-west line was drawn along the latitude of the average, and using our "surrogate crater" conceit, we searched to the east to identify a likely landform to investigate as an impact crater. Our first investigation target was Lake Michigan. When that structure was comfortably maintained as a glacially carved lake basin, we moved further east to the Saginaw Bay area of Michigan. Once identified, our numerical model was further enhanced to address the third geophysical flow function, the Systematic by Latitude conjecture.
Utilizing the Google Earth GIS enhanced the identification and cataloguing of ejecta and crater landforms. The annotation of each location with structural characteristics and linking of GIS information was accomplished, and the resulting database was managed as a structured metadata text file, easily distributed to other collaborative users. As we built the database of these elements, we offered public access to the "Keyhole Markup Language", or KML file through a thread of posts presented on the Google Earth Community, Nature and Geography (Moderated) Forum: Inferred Orientation of Distal Ejecta.
Most attempts at following the inferred orientation of the bays back up the trajectories' bearing have failed to produce a focus. We propose this to be caused by at least three large-scale geophysical flow properties not considered. First, that the impact may have been a shallow-angle-of-impact (oblique) event, which would infer a chaotic focus, and secondly, that the earth rotates during any realistic ejecta loft time, creating an orientation effect which we attempt to demonstrate with the attached kml file. A third variable is the proper accounting for the west-to-east ground-velocity vectors between the ejection site and the landing site, which will be resolved as the ejecta re-enters the lower atmosphere and strikes the earth. If a model could be created to replicate those large-scale geophysical flows, it should be able to replicate the as-found orientations of all Carolina bays. Further, if the model was built to generate a distal ejecta sheet emanating from our proposed impact site, those correlations could be considered strong support for the impact argument.
Our heuristically-derived model for the distribution of ejecta from the proposed impact can be tested by programming it to generate "predicted" orientations for a given Carolina bay location, and empirically comparing that orientation to one which is apparent from that particular Carolina bay's planform. The Google Earth GIS system was leveraged to visualize numerous locations that contain Carolina bays. Using the Google Earth "Placemark" metadata element, the location's latitude and longitude are captured and annotated. A web-based calculator was developed to process those placemarks and return a set of Google Earth elements that represent the numerical model's predicted orientation. The calculator can also reverse the process, and provide a "walk-back" to a putative crater by processing a user-adjusted Bearing Arrow as the input datum. In all cases, the data transfer is accomplished by using Google Earth's "Keyhole Markup Language" (KML), a dialect of XML specifically created to encapsulate geographic information system datum.
In addition to the interactive calculator, a spreadsheet was developed to act as a database repository for all bay field metrics. The spreadsheet generates both the predictions and the walk-back solutions, allowing for a simultaneous solution to all fields in the database against input variables such as proposed impact location, average ejecta velocity and terminal velocity. The spreadsheet also generates sets of Google Earth KML to visualize the model's output in the GIS tool.
3.3) "Systematic by Loft Time"
During the time period extending from the moment of the source impact to the eventual deposition of the distal ejecta, we see the de-coupling of the spatial coordinate reference systems in multiple dimensions. The decoupling is driven by the spherical nature of the "playing field" when trajectories cover significant distances. The common term applied to the effect is the Coriolis Force, which is a kinematic force applied to an object to "force" it along a great circle route as a object proceeds along its trajectory. For example, if an object is launched with sufficient velocity on an azimuth of 90 degrees from latitude 45 north (i.e. due East), it will follow a great circle route as it begins to "circle" the earth's spherical surface. The Cartesian coordinate "bearing" of our example object begins to "turn" south, and eventually the object will cross the equator on an azimuth 45 degrees increased, or 135 degrees. But the above analysis does not account for the fact that the earth is also rotating during any real-world loft trajectory period.
Using the Google Earth facility, we evaluate the effect on the Cartesian coordinate system of azimuths and bearings when the Earth's rotation is considered. During our modeled 12 minute loft time shown in Figure 6, the Earth will rotate three (3) degrees of longitude from the west to the east [regardless of your location on the earth]. Therefore, the landing location of the ejecta will actually be three degrees westward of the initial "target".
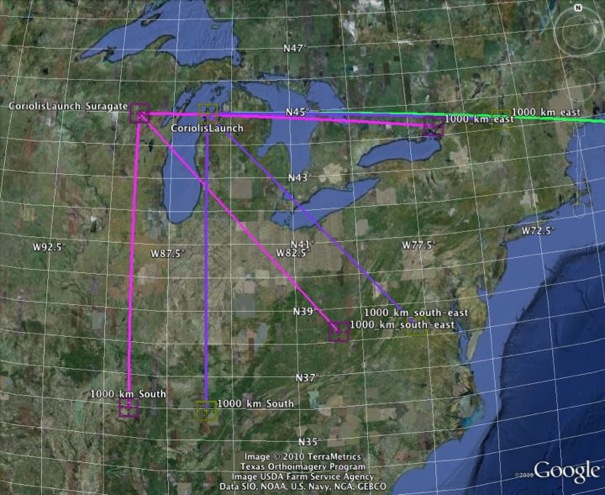

Figure 6 - Basic Example of Loft Time Rotational Offset (Google Earth set of kml linked)
When the ejecta is deposited at the eventual location, it will still bear the original flight azimuth/bearing. If those geometries are followed back along the trajectory, the will focus on a location three degrees west of the original launch location. Thus, we feel justified in applying the conceit that, from the perspective of the distal ejecta landing site, the inferred bearing would point back to a surrogate impact crater. The surrogate would offset on the global map by one degree of longitude westward for every four (4) minutes of loft time, thus we consider this effect to be "Systematic by Loft Time".
3.4) "Systematic by Latitude"
The Carolina Bays display a marked alignment with northwest-southeast being the preferred orientation. Although there are minor local fluctuations, deviations from the preferred orientation appear to be systematic by latitude (Prouty, 1952).
While the Coriolis force component is systematic by loft time, there is another factor superimposed on alignment that is systematic by latitude. In the first case, the earth rotates at 0.25 degrees of longitude per minute, regardless of where on the earth the consideration is applied. In our second case, we rationalize that the ground speed of any particular spot on the earth is a function of the cosine of its latitude. The end cases are the poles - where the ground velocity w>e due to rotation is negligible - and the equator - where the ground speed w>e is ~1,670 km per hour.
In our specific Saginaw Manifold, a relevant set of w>e velocities would be our Saginaw Centroid point - rotating at 1,270 km/hr - and a generic ejecta field such as Bishopville - rotating at 1382 km/hr. At time of contact the 165 km/hr w>e velocity difference will resolved by a skewing of the "splat", effectively rotating the inferred bearing in the clockwise direction.
By way of explanation, a droplet of ejecta traveling from the north to the south in its great-circle frame of reference would not be affected by that ground speed difference until it approaches the surface of the earth, where the atmospheric breaking effect on terminal velocity would be applied. Across those last momentS of the flight, the west-to-east ground velocities will be resolved. Given a point object such as a missile warhead, this resolution need not be considered. When an object as large as our posited ejecta droplets (say, 100m diameter) lands, this resolution will skew the inferred arrival bearing. In our cases the velocity difference needs to be subtracted from the inferred w>e velocity vector. Since the n>s velocity vector is constant, the effective alignment rotates clockwise slightly.
How relevant is this? It may be considered to be a minor factor in the more northerly fields, where there is less w>e velocity differential to detract form a relatively high w>e loft vector. In the more southern fields, the adjustment becomes a much higher factor both in magnitude, and in respect to the smaller w>e loft velocity vector. When superimposed on the counter-clockwise effect of the Coriolis (systematic by loft time), the latter may tend to overpower the former. We suggest that when moving north to south through the fields this general shift of the alignment vector clockwise is seen as "systematic by latitude". .
Figure 7 attempts to exhibit the numerical adjustments we make to the inferred alignment to predict a de-skewed arrival bearing at a particular field of bays. The graphic uses the metrics of Wagram, NC's field.
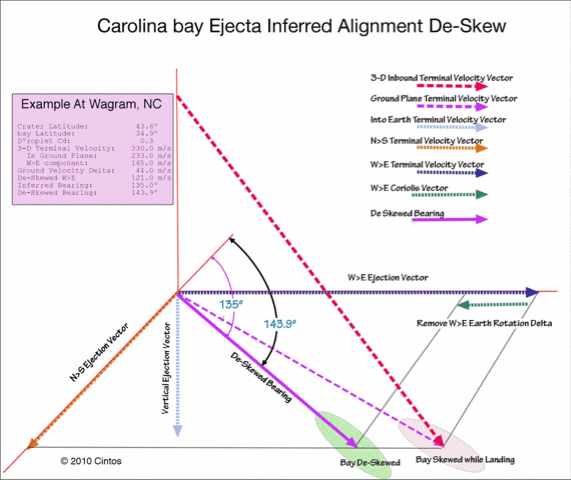
Figure 7 - De-Skew Arrival Bearing Calculation
Please consider, also, that the loft time is a variable affected by both the launch velocity and its loft angle. A trajectory can be generated for a given landing location using shallow lofts (and short transit times) as well as higher loftings which would take longer to get to the same location. And the results of both the loft time and skew factors? Chaos within a bound set of results.
Our discussion of the "Systematic by Loft Time" and "Systematic by Latitude" can be found int the Modeling Bay Orientations Chapter.
3.5 Bearing Calculator For Creation of Google Earth KML to visualize Inferred Alignments
A challenging aspect of our initial empirically-derived 22 minute loft time is not immediately supportable with a common trajectory model, which force a loft apogee that is unrealistically high for a skewed landing. At a more realistic 30 - 45 º, the loft time from the two available ballistic programs we have used are in the range of 400 to 600 seconds. The "Systematic by Loft" model was numerically extended by applying the "Systematic by Latitude" conjecture. The topic is discussed in more detail in the Bay Orientation section.
The numerical model developed here is considered by us to be very simple and elegant. The only variables being perturbed are the average velocity of the ejecta during its flight (with loft time as the tuning proxy) and the terminal velocity of the falling droplet of ejecta ( with the pellet's coefficient of drag Cd as the tuning proxy). At each field of bays, we extract the latitude and longitude as test case input constants. The model was heuristically focused on the Saginaw Bay proposed structure and it's three control points (NE, Centroid and SW); those values are similarly applied as constants across all calculations. The 140+ bay location "fields" represent many thousands of individual bays, and the solution sets are resolved against all fields simultaneously, with all bearings falling within the control points. While it would seem plausible that the ejecta at any given location may actually have a density or velocity different from other locations, the model did not have to leverage such fine tuning to arrive at simultaneous solutions for all 140+ fields
4.0 Results
Using the methods developed, we have identified a probable impact location. Predictions for Carolina bay alignments at a given field's latitude and longitude were generated using the numerical model, and were correlated with the empirically measured orientations. We demonstrate how our analysis of the sequences, orientation and geometry of the proposed crater and distal ejecta support our conjecture.
4.1 ) Identification of Cosmic Impact Location
Using an empirically measure inferred arrival bearing at about 40 Carolina bay fields, we numerically calculated great circle paths for visualization in Google Earth. A satisfactory triangulation locus was evident between the bays on the east coast and those in Nebraska. To enable visualization of the triangulation in Google Earth, we invoked the conceit of placing a surrogate "copy" of a large oblique crater outline at that locus. That value was further rounded for simplicity to a value of 43.5 North Latitudes and 89.5 West Longitude.We speculate that the direct great circle triangulation can only identify the actual impact site locus if the earth was not rotating during the ejecta loft interval. Given the earth's rotational rate, a loft time longitude offset effect will increase by ~ 0.25 degrees of longitude per minute of flight time. The modeled ejecta loft time of 4 to 24 minutes would generate a bearing offset to a surrogate crater location 1 to 6 degrees westward. Conversely, the actual impact crater should be located be along a path extending due east from the surrogate crater location. We heuristically examined various geological depression found along that transit, and have settled on evaluating the Saginaw Bay are of Michigan's Lower Peninsula (LP) for further analysis. Our proposed impact site at Saginaw Bay represents approximately a 22 minutes loft time offset (equating to 5.5 degrees longitude rotation) , which generates a working impact centroid at 43.58 North Latitude and 83.94 West Longitude.
Figure 8 presents a portrait of our current proposal for an impact site, as derived from the Coriolis-adjusted bearings to the Carolina bays. The yellow paths represent the empirically measured inferred alignments extended back along great circle lines from the individual fields to the surrogate crater. The green paths are computed great circle lines using a 5.5º eastward offset from each field to adjust for 22 minutes of earth rotation during the loft.

Figure 8 - Saginaw Bay Solution to Carolina bays' orientation, with focus back to a surrogate crater location.
4.2) Heuristically Modeling a Saginaw Bay Cosmic Impact
The flight lines, distances and bearings of all catalogued Carolina bay fields have been analyzed for correlation to the proposed surrogate impact crater site. Our first correlation considers the geographical great circle distance from each field back to the proposed impact centered at Saginaw Bay. As shown in figure 9, a very high degree of correlation is seen. The fields are ordered left to right as they are positioned clockwise around the crater location. This suggests several things. First, an ejecta curtain was lofted with a fairly consistent loft angle and velocity. Second, the distances do increases slightly as the landing fields progress radially along the butterfly pattern, suggesting that down-range ejecta was slightly more energetically lofted. Finally, Fields to the west are highly correlated distance-wise with their counterparts in the Southeast, across the ejecta free blow-out zone. The shortest distance seen are in the New Jersey & Maryland areas, which have no counterpart to the west owing to that section of the arc would have landed on the glacial ice sheet.
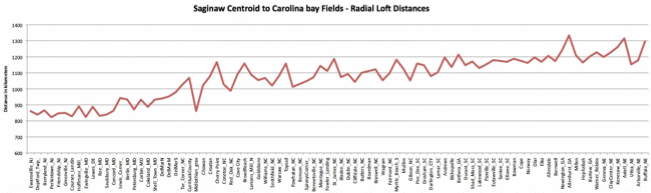
Figure 9 - Great Circle Distance between the proposed crater centroid and each of the evaluated Carolina bay fields.
The correlation above suggests that the bays are geographic distributed along a narrow and highly symmetrical pair of "butterfly" arcs centered on the triangulated Saginaw impact location. Such a distribution is suggested in much of the current research on oblique impacts. The expected down-range "no fly" zone is apparent in the distribution shown in Figure 10, which displays the arcs in Google Earth, and also demonstrates the symmetrical nature of their locations around the implied impactor's azimuth. We note that all areas north of the impact site were covered in glacial ice sheet, prohibiting formation of identifiable bays in those areas.
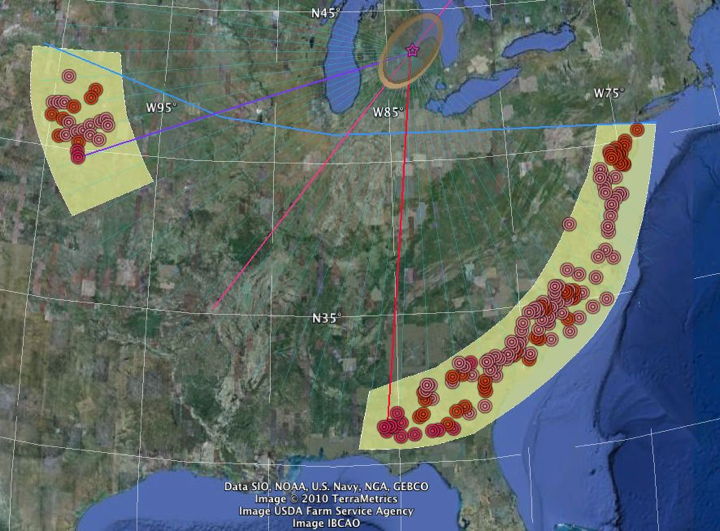
Figure 10 - Carolina bay locations distributed in butterfly arcs symmetrically around Saginaw Crater (Ice Sheet above blue line)
For each of the evaluated Carolina bay fields, we measured an inferred arrival bearing for the ejecta. These measurements can be validated by reviewing each field in Google Earth using the provided kml elements. Three sets of great circle bearings were generated from each Carolina bay fields back to 1) the surrogate crater centroid (43.5 N, 89.5 W); 2) a point to the NE representing the north-eastern limit of the crater ejection rampart ( 44.5 N, 88.6 W); and 3) a point to the SW representing the south-western limit of the crater ejection rampart ( 42.5N, -90.5 W). These great circle lines can be imported into Google Earth using the SaginawCoriolisBearings.kmz file.
The numerical model creates a set of three predicted bearings for ejecta landing at that location. The three bearings correlate to the oval Saginaw crater's centroid, and two outer rampart locations near the ends of the crater's major axis. The process is detailed in the Bearing Calculator - Point section of the website. Figure 11 presents results from our 140+ bays when the only provided datum is a single Google Earth Placemark point.
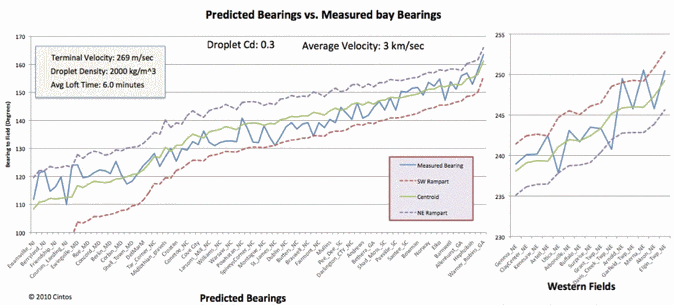
Figure 11 - Predicted Bearing Alignment Correlation Plot
Figure 12 presents the correlation results when our de-skewing algorithm is applied at a given location using a user-oriented "Bearing Arrow" as a bay orientation estimate. The process is detailed in the Bearing Calculator section of the website.
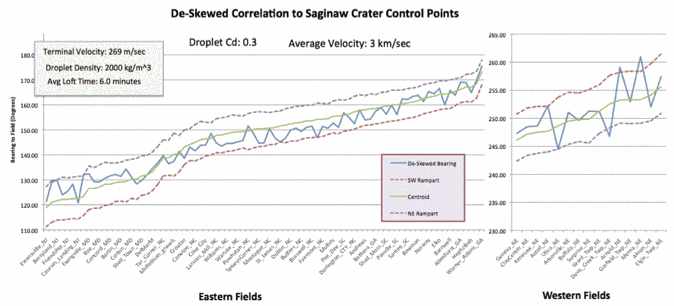
Figure 12 - De-skewed Alignment Correlation Plot
The plot in Figure 13 shows the sensitivity of the two input variables for a broad range of tested solutions in which all 140+ evaluate Carolina bay field sites correlated to the Saginaw crater site. We suggest that a realistic range for loft time is in the range of 6 to 10 minutes, as confirmed by ballistic modeling programs. We note the asymptote of the terminal velocity to ~300 meters/sec within that range.
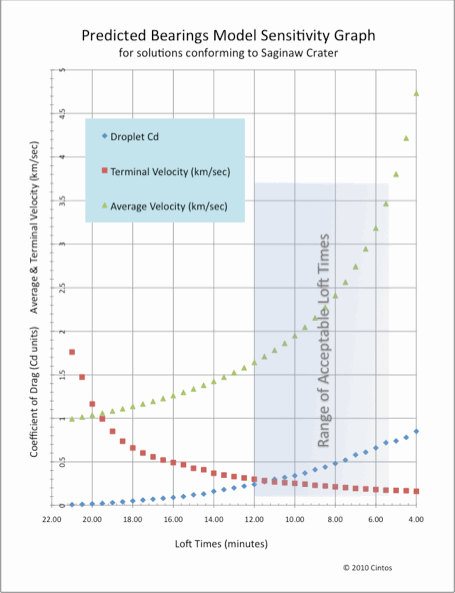
Figure 13 - Sensitivity Plot of Model Variables
6) Discussion
6.1) The Saginaw Impact Crater
With an identified and positively correlated impact site, we turn our attention to validating the proposed area of the Lower Michigan Peninsula. More detailed discussions are presented in the Saginaw Bay section of our site. We have identified a significant number of anomalies across the central lowlands of the Saginaw Bay area. We are quite aware of the long-standing acceptance that the area was scoured out by multiple advances and retreats of the Laurentide Ice sheets. Ironically, we are actively considering it based on the reason we dismissed the Lake Michigan area as an impact site: The bedrock across the central lower Michigan Peninsula is composed of later, more solidified carbonate rocks, whereas the older underlying rocks are softer shales and sandstones built up prior to the origins of life and the calcium deposits derived from shells. While the ice age glacial sheets were removing vast quantities of strata from around the basin's periphery, they were generally unsuccessful in breaching the carbonate layers towards the center of the basin, with one major exception - Saginaw bay.Unique landscapes - Unique Geological Enigmas
The Goldsboro ridge is a unique feature on the Sunderland surface and requires special explanation whatever its origin. It must be either an erosional remnant of a once more extensive sediment or a depositional feature. The Goldsboro Ridge, an Enigma, R. B. Daniels, E. E. Gamble, Wheeler, et al Dr. Daniels and his team went on to examine each of the two possibilities presented to them: sedimentary or depositional, without arriving at a satisfactory solution. The paper closes with the comment "But, until much more is known about all the middle Coastal Plain, the Goldsboro ridge will remain, in the last analysis, an enigma." The enigma continues today, and applies by extension to the vast swarms of Carolina bays, such as those lying imbedded in the brow of the Goldsboro Ridge.
Our hypothesis offers a truly "special explanation" for this continuing enigma. Would it not be proper that such a juxtaposition should exist in science? A cosmic impact "at our back door" in the Saginaw area can be argued, as that landscape is filled with geological anomalies. We discuss some of these anomalies in the Saginaw Bay Chapter. The Saginaw Bay watershed contains the largest contiguous freshwater coastal wetland system in the United States.[Saginaw River and Bay Area of Concern -U.S. Environmental Protection Agency]. Flowing out of this area of Michigan to the southwest , the Kankakee River and it's enigmatic torrent constructed what was at one time the second largest marshland and swamp in the US, after the Everglades (before man "fixed" it). Flow seems to hold sway in another historical precedence:
O-Sag-e-non or Sag-in-a-we from the Ojibwa language, which means "to flow out", is a possible origin for the name "Saginaw". Wikipedia® Entry
Confirmation of this area as an impact will likely take generations of research. Using visualization tools, however, we suggest there is a high correlation from a geometry perspective. Figure 14 presents the area in Google Earth, with two overlays: a color-ramp digital elevation mapping layer, and an appropriately sized and rotated oval shape file overlay. Why oval? Craters are circular, right??? Elliptical craters with "butterfly" ejecta patterns make up roughly 5% of the total crater population of terrestrial planets and Moons. They are caused by impactors which hit the surface at oblique, or very shallow angles, such as one from Mars discussed in the Unique Impact section.
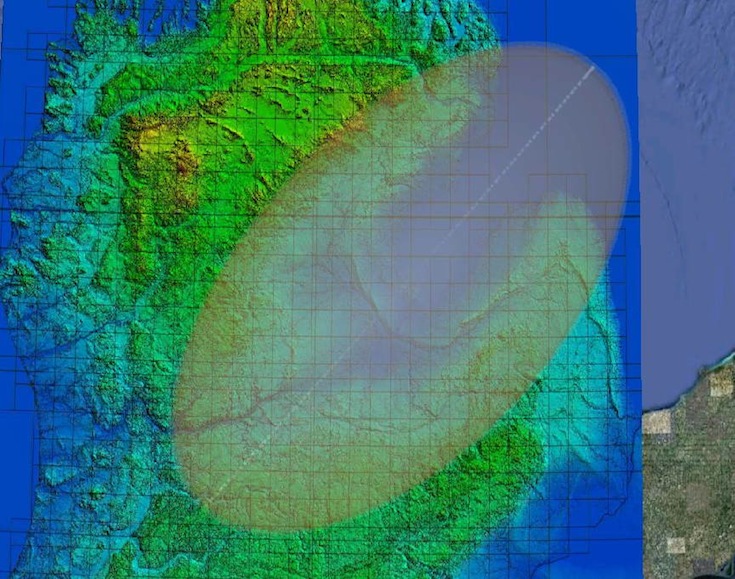
Figure 14 - Oval shapefile Overlay and DEM Image of the Saginaw Impact Site
We note the strong trend correlation across the northern and southern boundaries, as well as the overlap into the Kankakee Torrent flood plain in the southwestern end. Experimental and planetary imaging has identified oblique oval craters as typically displaying the deepest excavation at the up range end of the crater, which here falls in the Northeast directly over one of the deepest parts of the Huron basin - the Bay City Basin. From that point, the land rises gently across the peninsula, reaching its greatest elevation at the southwest end of the oval overlay. Another common attribute of an oblique impact is the existence of a slight ridge - likely rebounded strata - tracing al line down the center of the structure. In our situation, the Charity Islands rise up form the bay directly along the oval's centerline.
Please note that fully expect that the Huron lobe of the Laurentide Ice sheet would have eventually advanced at least 6 more times into and across the excavated crater from the Huron basin in the Northeast, bulldozing the collapsed 5km high ice crater ramparts, effectively resurfacing the region.
The database of evaluated bays continues to expand. Figure 15 depicts ~140 "de-skewed" trace-back trajectories yielding the Saginaw focus. This graphic can be reproduced in Google Earth with this file:
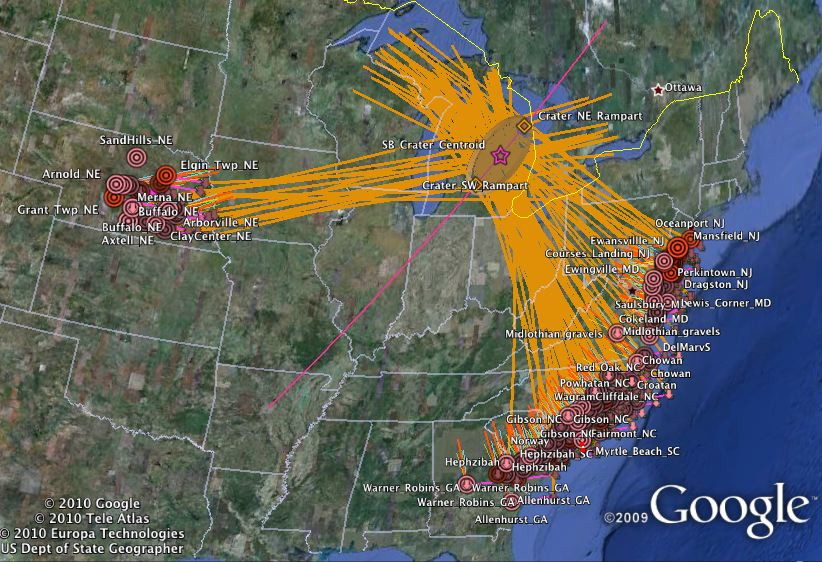
Figure 15 - Saginaw Predicted Trajectories De-Skewed from Measured Orientations
6.2) Timing of the Manifold?
While our initial manifold presentation focused on the YDB, please note that we have moved the proposed date of the impact back many thousands of years, to approximately 140,000 years ago. Based on our newest analysis, which correlates the Carolina Bay impact with the event which generated the Australasian Tektite strewn field, the data is now set at ~800,000 years ago. Dating this event will now require application of 10Be/26Al burial dating techniques. Our move is motivated by several constraints imposed by a Saginaw Bay impact.A necessary constraint implied in a catastrophic genesis for the Goldsboro Ridge is that a dating exercise must yield a unique age for the entire structure. By extension, that same date should be evidenced deep within all Carolina bay structures. The failure thus far to identify a unique date has been problematic for any catastrophic theory, and is used to defend a gradualist genesis. Without exception, the sands in question have failed to yield a date based on fossil evidence, as they are entirely unfossilifirous. Daniels’ granted a marine geomorphology to his Goldsboro Ridge deposit, but rightly mentioned in passing that the lack of fossil evidence was problematic for that solution.
Carbon dating of organic remains within the bowls of Carolina bays typically report ages beyond the method’s technological limit of 45,000 years. A database of ~50 OSL dates from bays and adjacent sand dunes has show them to have been deposited or reworked at ages from the Holocene back to over 120,000 years ago. I interpret all dates documented thus far to be minimum age constraints.
David R. Soller, in his 1988 US Geological Survey Professional Paper on the history of the Cape Fear River Valley, made a pertinent observation. He describes how the eastward flowing Cape Fear River has been incising its southwest channel wall episodically for over 2 million years, migrating laterally in that direction as its bed is titled by the pervasive uplift of the adjacent Cape Fear Arch. This process has resulted in a series of ever-younger terraces existing at incrementally lower elevations as the terrain is traversed from the backbone of the Arch towards the current Holocene channel and flood plain. Immediately above the current-era Cape Fear River flood plain is the Wando Terrace, dated by fossil and OSL dating to have been created during the Sangamonian interstitial, hence well prior to the last Glacial Maximum of the Wisconsinan Glaciation. Dates of 30,000 years ago to 100,000 years ago are given for this terrace’s sediments.
Moving further upslope towards the Arch, Soller documents the terrace of the Socastee Formation, created during the interstitial preceding the Illinoian Glaciation. This is dated as being created while the Cape Fear River occupied this surface 200,000 to 300,000 years ago. (Figure 4 & Figure 5). Soller’s rational for a 200 ka date for the Socastee Formation would need to be falsified to support a 800 ka event. In personal communications with the Dr. Soller, he dismissed his older dating estimates and stated that newer technologies must be sued. One sign of an older date (pre-800 ka) is shown in Soller chart below. In it, he suggests that the Cape Fear river migration must have accelerated 3 to 4 x during the Socastee and Wando paving events, compared to the previous 3 Million years. Not likelyy, I fear. In fact, it is more apparent that the river migration slowed once the climate moved to the current-era 100 ka glacial cycles, where sea levels dropped significantly compared to the previous shorter cycles. This would drive the river bed down 50 meters or more at low stand - that would lock the river to a channel that could not move faster against the progressively higher southwestern valley wall.
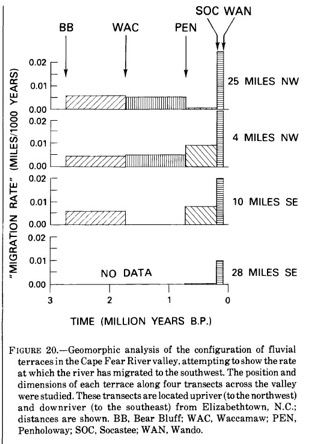
Considering dates for Carolina Bay formation, Soller makes the following statement of fact:
“Data gathered for this study provide a unique opportunity to indirectly date the formation of the Carolina bays and dunes. If it is assumed that all Carolina bays were formed by a single, unique climatic event, then this event must have occurred after deposition of the Socastee Formation and before deposition of the Wando Formation, as Carolina bays are prominent on the Socastee terrace but absent from the Wando. Since these terraces are correlated with isotopically age dated formations, the date of Carolina bay formation falls somewhere between roughly 100 and 200 ka, roughly equivalent to the Illinoian glaciation.”
Supporting this interpretation, the entire Socastee terrace is covered with impressively defined Carolina bays, right up to the abrupt scarp to the Wando terrace. Hence, the event would have been coeval with the position of the Cape Fear River when it deeply incised the terrain as a response to the significant lowering of the Atlantic because of the advent of increased glaciations in the 100,000 cycle that began at the time of the impact 800ka.
7) Conclusion:
Our hypothesis holds that ~800 kya a catastrophic impact manifold deposited a blanket distal ejecta up to 10 meters deep in a set of butterfly arcs across the continental US. We have modeled the blanket as a ballistically deposited hydrous slurry of sand and ice originating from a cosmic impact into the Illinoisan ice sheet, and propose that Carolina bay landforms were created during the energetic deflation of steam inclusions at the time of ejecta emplacement. In geographic areas presenting a favorably level hosting terrain and a relatively high water table, the paleobasin foundations of the bays were found to have persisted over the intervening millennia, in spite of being overlain with loess and subjected to water and wind erosion and reworking.
A numerical model was developed to predict the expected trajectories and emplacement orientation characteristics of the hypothetical ejecta blanket, and was expanded to "reverse" the geophysical flows, such that a given bay’s empirically measured orientation could be used to generate a "walk-back" triangulation trajectory to validate the impact site identification within Google Earth’s GIS viewer.
We presented evidence that, when applying a unique combination of impact location (Saginaw), average ejecta curtain wall velocity (3 km/sec) and ejecta terminal velocity (2.7 km/sec), our numerical model’s prediction of any given bay’s orientation correlates well with the empirically measured orientation of that bay. Significantly, the correlation is seen at all ~45,000 Carolina bays in our catalogue. We also note that the distribution of bays is highly symmetrical around the proposed impact’s azimuth.
As the model was researched, its progress was shared with the Google Earth Community through a thread, Inferred Orientation of Distal Ejecta. To facilitate independent testing of the hypothesis, a web-based version of the model has been made available at http://cintos.org/java/PredictBearings .
The authors maintain that the correlations presented here show that a unique geospatial relationship exists between all known Carolina bays and the Saginaw region, a result that presents a strong argument for the ejecta blanket manifold hypothesis.
Further research is proposed to investigate - in the context of our hypothesis - the true geomorphological nature of Michigan’s Lower Peninsula and of the enigmatic Carolina bays.

Geological Research by Cintos is licensed under a Creative Commons Attribution-NonCommercial-ShareAlike 3.0 Unported License.
Based on a work at Cintos.org.
Permissions beyond the scope of this license may be available at http://cintos.org/about.html.